ABSTRACT
Mark D. Ryckman, P.E., BCEE
Larry K. Seabolt, Jr., CHMM
Remtech Engineers, May, 2019 ©
Bioremediation is an efficient technology when compared to alternative technologies. The two major advantages are lower capital cost and the ability to perform the task insitu. Beginning around 2000, enhanced biodegradation, or biostimulation (stimulation of native heterothropic oil degrading bacteria), became widely accepted as a leading treatment technology. Many effective biostimulation supplements have been demonstrated and include the addition of surfactants, nutrients, enzymes, electron donors, electron receptors, and carbon substrates (1).
The purpose of this treatise is to 1) provide an understanding of bioremediation’s key mechanisms, 2) provide optimization techniques and sample delivery methods, 3) present work flow diagrams for different matrix applications, and 4) present case histories for petroleum hydrocarbon bioremediation in soil and groundwater (Figure 17), railroad ballast (Figure 18), and gravel/soil at power plants and electrical substations (Figure 19) for a natural non-toxic bioremediation accelerant, HC-2000.
Authors
Mark D. Ryckman, P.E., BCEE
Mr. Ryckman has served as principal engineer on over 10,000 environmental remediation projects projects during the last 40 years. Mark D. Ryckman founded Remtech Engineers in 1988 that specializes in soil and groundwater remediations. He is a Registered Engineer in seven (7) states and is a Board Certified Environmental Engineer in Water & Wastewater and Hazardous Waste Management and Environmental Remediation (HW) by the American Academy of Environmental Engineers and Scientists. Prior to starting Remtech, Mr. Ryckman served as Executive Vice President of D. W. Ryckman & Associates/REACT with his father Dr. D. W. Ryckman (MIT graduate). Dr. "Rick" Ryckman founded one of the first Graduate Environmental Engineering programs in the United States at Washington University in St. Louis, Missouri. Mr. Ryckman possesses a B.S. in Civil Engineering and M.S. in Environmental Systems Engineering from Clemson University and a B.A. in Mathematics from DePauw University.
Larry K. Seabolt, Jr., CHMM
Mr. Seabolt has served as Vice President of Remtech Engineers for over 26 years and possesses an engineering degree from Kennesaw State University in Civil & Environmental Engineering. He is a Certified Hazardous Materials Manager, and has chaired the Operations Committee of the GA DOT TIME (Traffic Incident Management Enhancement) and Cobb County Resource Council (LEPC). Mr. Seabolt has served as project principal on over 8,000 multi-media environmental projects and is a ITS Qualified Operator (Integrated Transmission System) for Power Substations Damage Assessments & Switching, Certified Safety Trainer for Norfolk Southern and CSX Railroads, Hazwoper, and numerous OSHA, EPA, DOT, Safety, and Hazardous Material Training Courses.
1. Introduction
Completing a significant number of soil and groundwater remediation projects to very low limits has in many cases been technically and financially unachievable. Underlying issues include: lack of understanding of the complex engineering and scientific subsurface biological, chemical, and physical processes; difficulty in quantifying contaminant mass transport through a variable and limiting subsurface cross section; underestimating the impact of contaminant sorption and rebound (back-diffusion); lack of experience with recalcitrant environmental contaminants such as Dense Non-Aqueous Phase Liquids (DNAPLs), fuels, and chlorinated solvents; and lack of funding (1, 2, 3).
Risk-based solutions that leave some of the contamination behind (or rendering them immobile to impact downgradient receptors), while protecting public health and safety, are becoming more prominent and cost-effective. Solutions often involve removal, control, containment, or concentration reduction of the mobile/soluble phase to facilitate natural biological attenuation. This paper deals primarily with bioremediation of refined petroleum hydrocarbons rather than crude oil in fresh water environments. However, some of the same approaches may be utilized on crude oil in saline settings.
Bioremediation is an efficient technology when compared to alternative technologies. The two major advantages are lower capital cost and the ability to perform the task insitu. Beginning around 2000, enhanced biodegradation, or biostimulation, became widely accepted as a leading treatment technology. Many effective biostimulation supplements have been demonstrated and include the addition of surfactants, nutrients, enzymes, electron donors, electron receptors, and carbon substrates (1).
The purpose of this treatise is to 1) provide an understanding of bioremediation’s key mechanisms, 2) provide optimization techniques and sample delivery methods, 3) present work flow diagrams for different matrix applications, and 4) present case histories for petroleum hydrocarbon bioremediation in soil and groundwater (Figure 17), railroad ballast (Figure 18), and gravel/soil at power plants and electrical substations (Figure 19) for a natural non-toxic bioremediation accelerant, HC-2000.
2. Understanding Bioremediation
Biodegradation is defined as destruction of a chemical by the action of living organisms (4). Bioremediation uses microbial metabolisms in the presence of optimum environmental conditions and sufficient nutrients to breakdown contaminants, notably petroleum hydrocarbons. Bioremediation efficacy hinges on site specific requirements such as the availability of sufficient quantities of microorganisms capable of degradation, nutrient availability to support microbial growth and proliferation, contaminant concentration and type, contaminant location, and environmental parameters including temperature in sufficient contact time for treatment.
Bioremediation involves the production of energy in a redox reaction within microbial cells. These reactions include respiration and other biological functions needed for cell maintenance and reproduction. A delivery system that provides one or more of the following is generally required: an energy source (electron donor), an electron acceptor, and nutrients. Different types of microbial electron acceptor classes can be involved in bioremediation, such as, oxygen, nitrate, manganese, iron (III), sulfate, or carbon dioxide, and their corresponding redox potentials. Redox potentials provide an indication of the relative dominance of the electron acceptor classes (5). Cometabolism is another mechanism involved in the breakdown of contaminants. Cometabolism is defined as a biotransformation when microorganisms use other carbon sources as their primary substrate rather than the contaminant to produce enzymes that degrade contaminates.
BIOREMEDIATION DESIGN OPTIMIZATION PRIMER WITH HC-2000
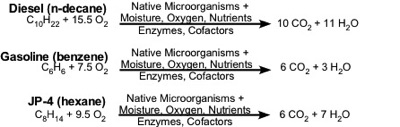
2.1 Role of Native Microorganisms
The majority of soil and groundwater petroleum hydrocarbon contaminated sites contain a sufficient consortium (mixture) of microorganisms (bacteria, fungi, yeast) capable of degrading most contaminants (5, 6, 7, 8). Multiple native organisms are present in the aerobic, facultative, anaerobic, methogenic, and fermentative zones that all contribute to the mineralization of organic contamination. No single organism can complete the process. What is generally missing are the complete conditions necessary for indigenous species to function effectively. Specific requirements include nutrients, growth substrate inducers, electron donors, and electron acceptors. Most of the native bacterial species in soil remain unidentified due to limitations in existing microbial identification methodologies. The mere presence of documented petroleum degraders may not be enough to ensure degradation. Less than 1% of environmental isolates are cultural and therefore do not accurately represent the actual community of hydrocarbon degraders (8).
Native soil populations capable of degrading petroleum hydrocarbons have been reported to range from 10 to 20% of all soil heterotrophic bacteria. In railroad ballast, 59 to 94% of all organisms are petroleum hydrocarbon degraders. Soil particles containing heterotrophs are found in lower concentrations in groundwater and surface water (2 x 104 cells/ml to 2 x 106 cells/ml) than in soil (2 x 107 cells/gm) (9), but provide enough metabolic and synthetic activity to digest petroleum hydrocarbons. Approximately 67% of all bacteria in groundwater sediment are attached to the silt and clay fraction (3).
2.2 Microbial Assimilation
Native communities, which are exposed to hydrocarbons or pollutants, become adapted, exhibiting selective enrichment and genetic changes where contamination becomes their source of food. The adapted microbial communities can respond to the presence of hydrocarbon pollutants within hours and exhibit higher biodegradation rates than communities with no history of hydrocarbon contamination (Figure 1)(5).

Figure 1: Microbial Adaption to Contamination
2.3 Understanding and Overcoming Limiting Factors
Petroleum and chlorinated hydrocarbon biodegradation in soil and groundwater can be limited by many factors including nutrients, pH, temperature, moisture, oxygen, soil properties, contaminant type, and contaminant location. Biostimulation involves the modification of the environment with limiting additives to stimulate existing bacteria. This can be done by the addition of various forms of limiting nutrients and electron acceptors, such as phosphorus, nitrogen, oxygen, or supplemental carbon (Figure 2). The primary advantage of biostimulation is that bioremediation will be performed by native microorganisms that are already present, distributed spatially, and adapted to the subsurface environment (5).
2.4 Overcoming Mass Transport Limitations
In order for bioremediation to take place, contaminants need to be transported to where microorganisms are most abundant, i.e., on fine soil particles. Since up to 60% of contaminants may be sorbed to soils, biosurfactants assist in the desorption and transfer of contaminants from larger to smaller particles in the unsaturated and saturated zones where degraders are present (Figure 3).
Seventy-five to 90 percent of contaminant transport occurs within 10 to 20% of the aquifer cross section. Consequently, the velocity of contaminants is higher than previously predicted when passing through a limited area (1). Mass transport and uniform mixing are primary limiting factors to bioremediation. Soil and groundwater are generally heterogenous matrices that pose uniform mixing and transport challenges due to highly variable transport velocities and mass fluxes. Treatment zones need to be identified for technologies to come in contact or mix with contaminants and the environment to be effective (2).
Mass flux is defined by the mass per unit time per unit area that a contaminant plume is transported through a defined cross sectional area. Mass discharge is expressed as mass per unit time. Collecting mass flux information through three-dimensional data gathering techniques can provide information to better target contaminants in their respective zones. Tools for collecting this data include: electrical conductivity meters, pH probes, dissolved oxygen sensors, cone penetrometers, direct push lithology evaluations, oxidation/reduction potential, and passive flux meters (1).
Simplified Microbial Aerobic Weathering/Mineralization of Petroleum Hydrocarbons